Bryony Lanigan is a Research Postgraduate in the Department of Physics. In this blog post, she shares more about her research as part of QuEST (Centre for Quantum Engineering, Science and Technology at Imperial College London). Bryony’s research aims to look for any hint of a dark energy candidate called the chameleon field.
What led you to study quantum research?
I don’t come from a family of scientists, but I do come from a family who have always encouraged me to do what I wanted. I’ve always loved learning – I was always that annoying child who couldn’t stop asking ‘but why?’ – and as it turns out, you can make a career out of that in research !
During my undergraduate degree, I took part in several short research projects in a wide range of fields, including acoustics, particle physics, and silicon quantum computing, and I enjoyed them all! When I decided that I wanted to pursue a PhD, I wanted to make sure it was something that had applications to a wide range of areas, and atom interferometry certainly fits the bill. I love experimental physics in particular because it’s so hands-on, and you have to understand concepts not just on a theoretical level but also the real-world effects and how they will impact your measurements.
Can you tell us about your research area?
I work with atom interferometers that exploit the quantum properties of matter to probe tiny changes in the environment.
First, clouds of Rubidium-87 atoms are laser-cooled to fractions of a degree above absolute zero. Temperature is related to velocity, so laser cooling atoms use lasers to slow atoms down. In one dimension, we can picture atoms moving along a laser beam, where the frequency of light is chosen such that if the atoms are travelling in the opposite direction to the light, the atoms will absorb photons before re-emitting them in a random direction, getting a tiny momentum kick backwards from each photon in the process. Do this with enough photons and in all three dimensions, and you can slow your atoms down and eventually trap them using magnetic fields in the centre of a vacuum chamber in a magneto-optical trap (MOT). This technique, along with methods to cool atoms even further, won the Nobel Prize in 1997. [1]
To use our atoms to probe their environment, we release them from the MOT and pump them all into one energy state. We then use carefully timed laser pulses to put each atom into a superposition of two states which follow different trajectories but recombine at the end when we read out their final state. An important element of this is that it is not the cloud that’s in a superposition – each individual atom is in its own superposition. Any difference between the two paths will be reflected in the final state of the atoms, and by comparing our measured final state to theoretical expectations, we are able to calculate what forces affected our atoms over the course of the interferometer.
What are the main aims of your current research?
The aim of my research is to look for any hint of a dark energy candidate called the chameleon field. Dark energy drives the accelerating expansion of the universe, and while we see evidence for it in large-scale galactic surveys, we find no evidence for any local effects. There are many (many, many) theories that attempt to resolve these discrepancies, but we focus on the chameleon field.
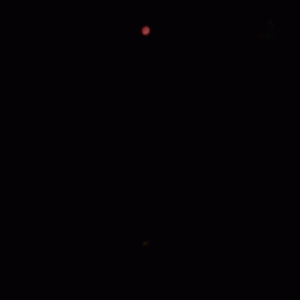
The chameleon field suggests that there is a field that is suppressed by the presence of mass, meaning that in our matter-dense local environment, it is forced to zero, but in the vacuum of intergalactic space, it is free to take on some high background value, pushing space outwards. Testing this is difficult – we’ve barely sent probes out of our own solar system, let alone into intergalactic space! This is not because we can’t create a good vacuum on Earth (we can), but how are we supposed to measure something that is suppressed by the presence of stuff? What do we use to measure it? This is where cold atoms come in: because atoms are so tiny and so not-dense, they would barely suppress such a field but are still affected by it. [2]
To attempt to measure the chameleon field, we have cold atoms inside a vacuum chamber, along with a ball of aluminium. Away from the ball, in the vacuum chamber, the chameleon field will be high, but on the surface of the ball (and inside it) the chameleon field will be forced to zero, meaning that in the region near the ball, there will be a gradient in the chameleon field, and so the atoms would experience a force.
With our interferometer, we measure the acceleration experienced by our atoms, and so we look for any extra acceleration that could be due to the chameleon field. It’s not quite as simple as this makes it seem – we need to control any potential source of error that could show up as acceleration very carefully, as we are trying to detect anomalous acceleration that’s less than a billionth the size of acceleration due to Earth’s gravity – and it takes years to build an experimental setup and characterise it so we can understand any measurements we take.
How could this research potentially benefit society?
At its base, my experiment uses atoms as probes to sense tiny changes in acceleration, and so can be applied to sensing other kinds of accelerations – you just need to find the right way of measuring it. Inertial navigation is something that others in my research group work on, and it uses the same fundamental principles that my research does. Using cold atoms as quantum sensors for navigation could provide an excellent alternative to global navigational satellite systems (GNSS) that could be used in places where GNSS is unavailable, e.g. underground, or under the ocean.
What are the next steps in your research? Are there any challenges ahead?
There are always challenges ahead, that’s one of the best things about research! Currently, we are trying to iron out the last few problems before taking measurements to try to find evidence of the chameleon field – or, rather, find no evidence of the chameleon field.
Other experiments that have been done have yet to find any evidence of anomalous acceleration that could be linked to a chameleon field, and we are searching right down at the tiniest edge of accelerations. In this field, we spend a lot of time measuring zero, but that’s still progressing science – and we’re still learning a lot about atomic and laser physics as we go.
[1] https://www.nobelprize.org/prizes/physics/1997/summary/
[2] https://iopscience.iop.org/article/10.1088/1475-7516/2015/03/042