Solar energy can be used to convert CO2 and methane, potent greenhouse gases, into high-value products for the production of fertilizers, plastics or even pharmaceuticals. In this post we find out about the materials needed catalyse this conversion.
By Dr Miriam Regue and Dr Minsu Park, Research Associates in the Department of Chemical Engineering.
Solar fuels have the potential to help us reach net zero carbon emissions. But they can also play an important role in the making of new carbon-based products following a sustainable circular economy approach.
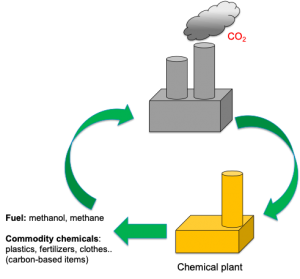
However, despite great promise, solar fuels are still far from being commercialised. But why? What is hindering their deployment, and what limitations are we facing?
The ideal solar material
It is both hard and challenging to give just one simple answer to this question. Remember from our first post that we said certain materials can absorb the energy from sunlight and transform it into electrical current? The right light absorber materials for a transition to a solar fuel-based society are still being researched. In fact, the “ideal” light absorber must fulfil several requirements. First, it must absorb the widest possible range of the solar spectrum, so we can make the most of the solar energy received. Secondly, it must be made of elements with a plentiful supply on the Earth’s crust.
Solar materials and the radiation spectrum
To understand the first requirement, we must consider the solar spectrum. It is composed of three different bands: infrared, visible and ultraviolet radiation. Infrared and visible radiation are the majority bands, accounting for 52–55% and 42–43% of the spectrum respectively, whereas ultraviolet radiation represents only 3–5%. Infrared radiation, the largest band in the solar spectrum, is not very energetic. This makes it very difficult for activating the light absorber material and triggering the production of solar fuels. Therefore, light absorber materials can mainly be activated by either visible or ultraviolet radiation. The “optimum” light absorber material should be particularly good at absorbing light from the visible range (400 – 700 nm).
Each material has a specific and unique absorption range – called band gap energy. The band gap energy and the absorption energy are indirectly related. The higher the absorption wavelength, the lower the band gap energy. The band gap values that correspond to the visible wavelengths of the solar spectrum are 1.9 to 3.1 eV.
Solar materials and elemental abundance
The second requirement is our light absorber material must be made of elements with a plentiful supply on the Earth’s crust. These are the elements coloured in green in The Element scarcity periodic table.
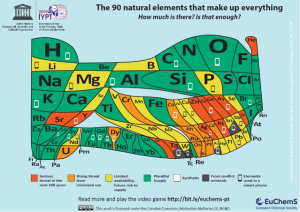
Haematite is ideal! Or is it?
Haematite (α-Fe2O3) is a promising light absorber material. It has a band gap energy of 2.1 eV, so it can absorb near the range of 590 nm. It is made of large abundant elements, iron (Fe) and oxygen (O). This makes it fulfil both our requirements!
However, haematite’s inherent properties are still not good enough to produce solar fuels in an efficient manner. Ultimately, the efficiency of the process also depends on several inherent features of the light absorber material. These include their energetic properties and their ability to convert the absorbed solar energy to the desired solar fuel. These are the most challenging features to control. The research community agrees that these are probably the limiting step of the solar fuel production. Finding the proper material able to meet all these requirements at once is a cumbersome process. It requires the collaboration of scientists and engineers from different fields of expertise such as theoreticians and experimentalists.
If existing materials such as haematite or TiO2 are not good enough for solar fuels, how can we improve them? Read our next blog about how can we find novel materials with more suitable properties. Or read our first blog in this series about the potential of solar fuels to produce clean green hydrogen.