Over summer, IMSE welcomes undergraduate students to spend a day working side to side with our Operations team. They help us explore new topics in the field of molecular science and engineering and draft questions for upcoming podcasts. In July, Polly Dean (Biology) and Manya Bhargava (Physics) combined their interest in plant science, quantum physics and genetics to write a blog exploring the interaction between these fields and the wide range of applications.
Quantum mechanics is a branch of Physics which describes the behaviour of subatomic particles (electrons, protons, neutrons). Genetic engineering is a method in Biology research which alters an organism’s characteristics by manipulating its genetic material. Despite their different definitions, both influence processes that control molecular interactions. Bringing together their knowledge on Biology and Physics, Polly and Manya explored examples where the combination of both disciplines (quantum and genetics) is solving global grand challenges. These include applying molecular aspects of photosynthesis to renewable energy systems, and increasing our understanding of immune responses for vaccine development.
Quantum coherence in photosynthesis
Photosynthesis is a biological process that takes place in plants, algae and some bacteria. It involves the conversion of light energy into chemical energy transforming water, CO2 and minerals into oxygen and organic compounds. The process occurs in chloroplasts where light harvesting complexes transfer energy to reaction centers through quantum coherence.
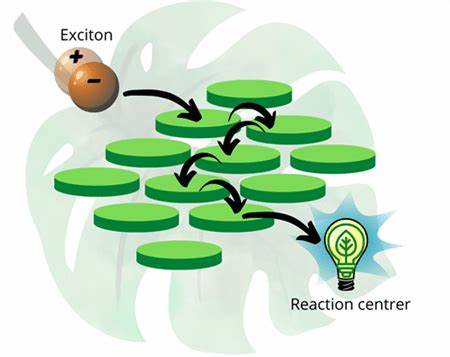
Quantum coherence is the process where small particles like excitons (energy carriers) exist in multiple pathways at the same time. Because of this, excitons are able to find the most efficient route to the reaction centre quickly reducing energy loss and maximising energy transfer.
Scientists have succeeded in mimicking the phenomenon of quantum coherence into other applications. For example, by designing artificial light harvesting systems with a high energy efficiency for use in solar energy plants. Additionally, researchers have genetically engineered plants to have the most optimum exciton route. This process has been used to produce fast growing crops, which are used to feed a human population with an increasing demand and population size.
Solar plant farm. Image credit: Heide Schwarze.
Quantum vaccines
As highlighted in our previous blog, vaccines have save millions of lives worldwide. However, developing them for some infectious diseases, such as tuberculosis and malaria, remains a challenge due to the complexity of these pathogens. Normally, scientists produce a weaker version of the virus, called an attenuated virus, which triggers an immune response from the body to produce cells targeted against the virus (T-cells). These T-cells recognise and attach to a part of the attenuated virus called the epitope. The vaccine epitope is identical to the real virus meaning that, when an infection occurs, the immune response is quickly activated.
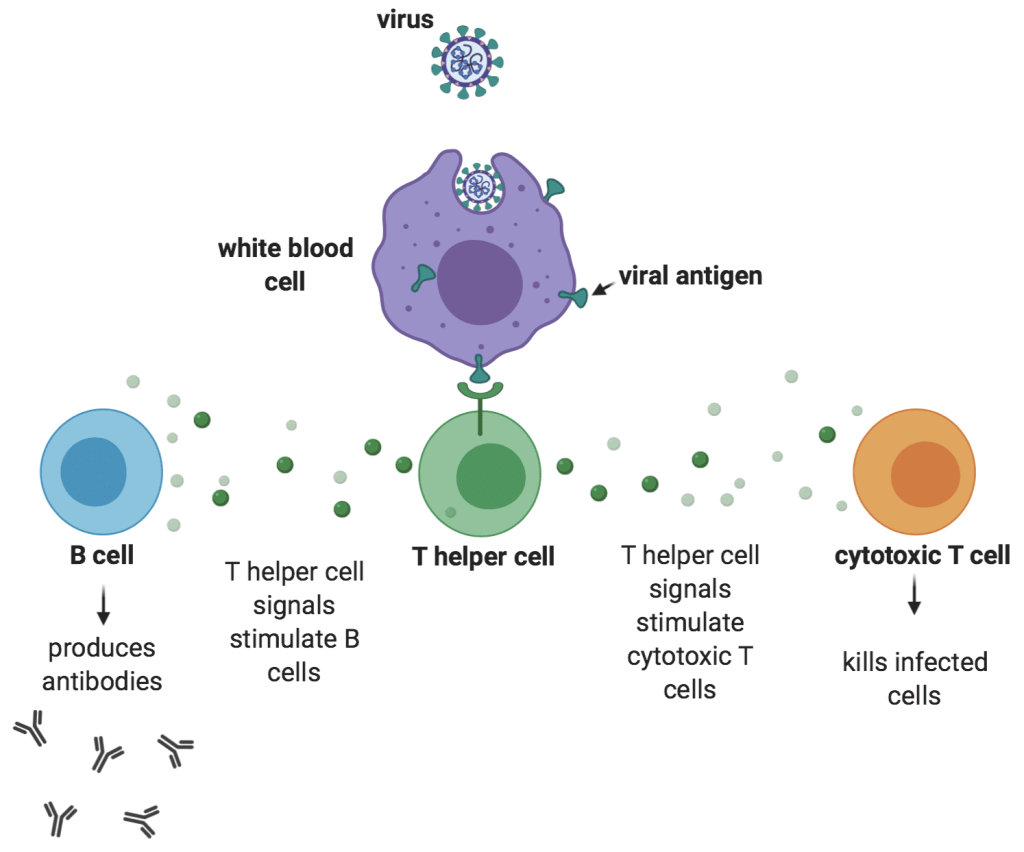
Quantum genetics, the study of underlying particle interactions within DNA, can also play a role in vaccine development. Quantum vaccinomics involves using our quantum genetics knowledge to produce algorithms that design T-cell epitopes in a more efficient manner.
Vaccines work through molecular interactions. Quantum immunology is the field that studies the signature of these interactions during immune responses. Similar to how the photon is called a ‘quantum of light’, immune protective epitopes are called ‘immunological quantum’. An additional strategy for vaccine development provided by quantum immunology is the analysis of epitopes to design protective antigens. This method has already been used to identify epitopes for different tick species. In the future, it has the potential to help design antigens with improved protection against multiple tick species in the same host.
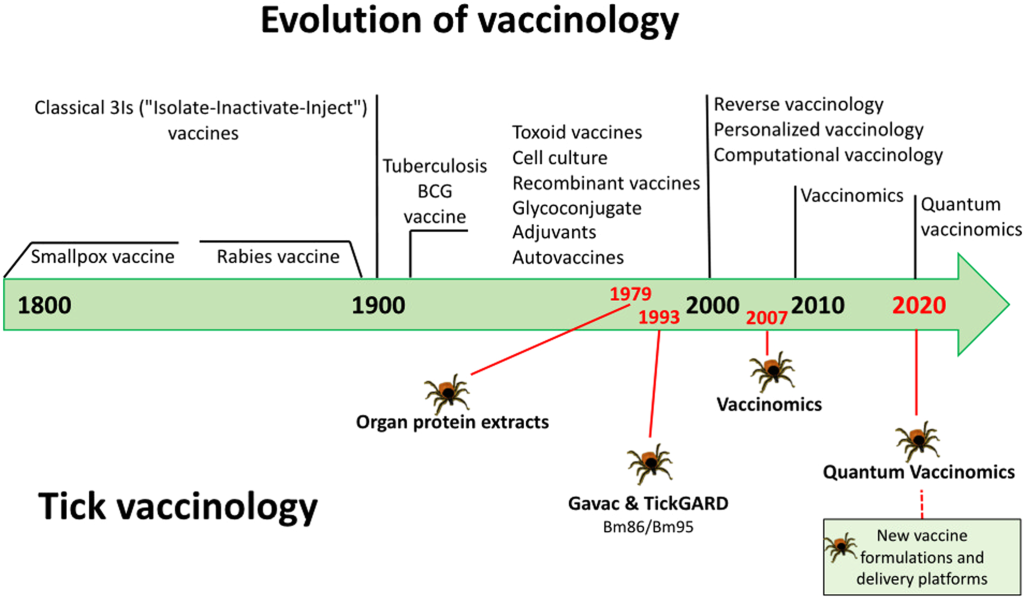
From molecular interactions to solving grand challenges
Both applications start at the molecular level (quantum mechanics and genetic engineering) and are scaled up through engineering to solve grand challenges.
Although at first physics and biology seem to have little to no overlap, the intersection of quantum mechanics with molecular and genetic engineering provides a promising outlook for the future of renewable energy and healthcare. From the use of genetically modified cells in lasers, to quantum technology in medical implants, the collaboration between these fields has a lot more to offer. The symbiosis of quantum physics and biology is a modern and relevant area of research. Interdisciplinary collaboration is the key to unlocking creative and innovative solutions to challenges facing us today. For us, it is the potential for advancements in technology, energy, and medicine, which drives our passion for this field. For example, in the future, quantum biology could help with the production of environmentally friendly materials, and sustainable technologies, important issues for our generation.