What concrete is to civil engineers, DNA is to biological engineers. At first glance, DNA might seem like any other material, a building block, like concrete. A material that, over time, humans learnt to refine, assemble, and optimize into products with specific functions. This analogy, brought to us by our guest blogger, MRes student Emile Durand, captures the essence of biological engineering’s approach. But to fully understand the potential of this topic, we must first learn what makes DNA different from other building blocks!
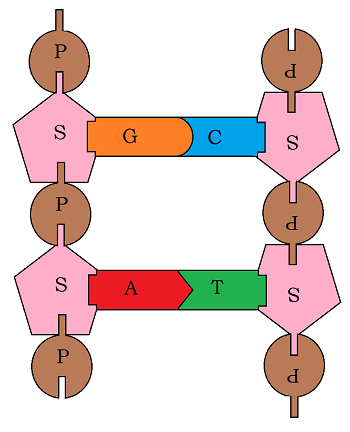
Consider something as routine as cell division: every day the cells in an average adult human divide roughly 1 trillion times. Each division is guided and coordinated by a few 600 or so proteins. These proteins need to adopt a slightly different structure to achieve their unique function during cell division. How do these proteins appear in the right shape and order, and at the right time a trillion times per day? DNA.
DNA is not only the concrete required to build a bridge, but also the blueprint that specifies assembly and design. Engineering DNA means being able to read, modify, fine-tune, and perhaps even invent new biological functions!
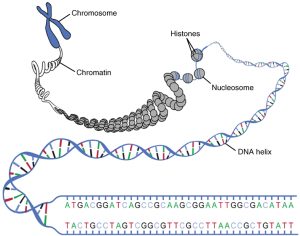
How does synthetic biology look like in practice?
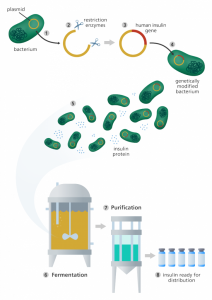
DNA engineering, also known as synthetic biology, began with a simple goal: turning cells into autonomous factories. In 1982, the pharmaceutical company Eli Lilly produced insulin through an engineered E. coli strain, a microbe commonly found in the intestines. They achieved this by introducing the human insulin gene into the E. coli’s own DNA, thus forcing the microbe’s genetical machinery to produce insulin.
This approach of combining DNA from different sources earned the name of “recombinant” technology. It marked a turning point in the pharmaceutical industry’s dependence on synthetic biology. The human recombinant insulin market reached 28.5 billion USD in 2024, while the global recombinant DNA technology market was valued at 730 billion USD in 2023!
In both academia and industry, the manufacture of proteins from recombinant cells raises a range of engineering considerations. “How can cells be genetically designed to maximize their secretion of products?” “How can we operate reactors to optimize cellular productivity?” “How can recovery processes maximize recovery yield without compromising product integrity?” “How can a product’s DNA be designed to facilitate its integration and production in the host?”
Applications
Nowadays, synthetic biology is expanding into new applications. Recently, excitement within the field has shifted from making cell factories, to directly applying DNA-based technology to improve health conditions. The all-too familiar mRNA vaccines are a perfect example of this. mRNA, DNA’s chemical cousin, was engineered to be a biological USB-drive, with enough information to train our body’s immune system against COVID-19. And, ever since the elucidation of CRISPR’s groundbreaking ability to selectively modify DNA in living organisms, the scientific body surrounding gene therapy has skyrocketed.
While this blog started highlighting the pharmaceutical applications of synthetic biology, the technology’s versatility is reaching several other industries. Biofuels? DNA engineering almost single-handedly provided the fundamental cellular tools to convert diverse feedstocks into bio-based carburant. Sustainable materials? Prof. Koon-Yang-Lee, along with Prof. Tom Ellis at Imperial & Modern Synthesis, are researching ways to engineer bacteria to sustainably produce leather.
Its growing relevance in numerous sectors, coupled with novelty and untapped potential, makes this field one to watch out for. Stay tuned for a series of blogs featuring how engineering biology is helping researchers solve grand challenges!