Ioanna Morianou recently completed her PhD (MRC DTP), at the Department of Life Sciences (Crisanti Lab). Here, she talks about her work as R&D Team Lead at Biocentis, innovating genetic tools to control agricultural pests, as well as the genetic strategies she utilised to help fight malaria.
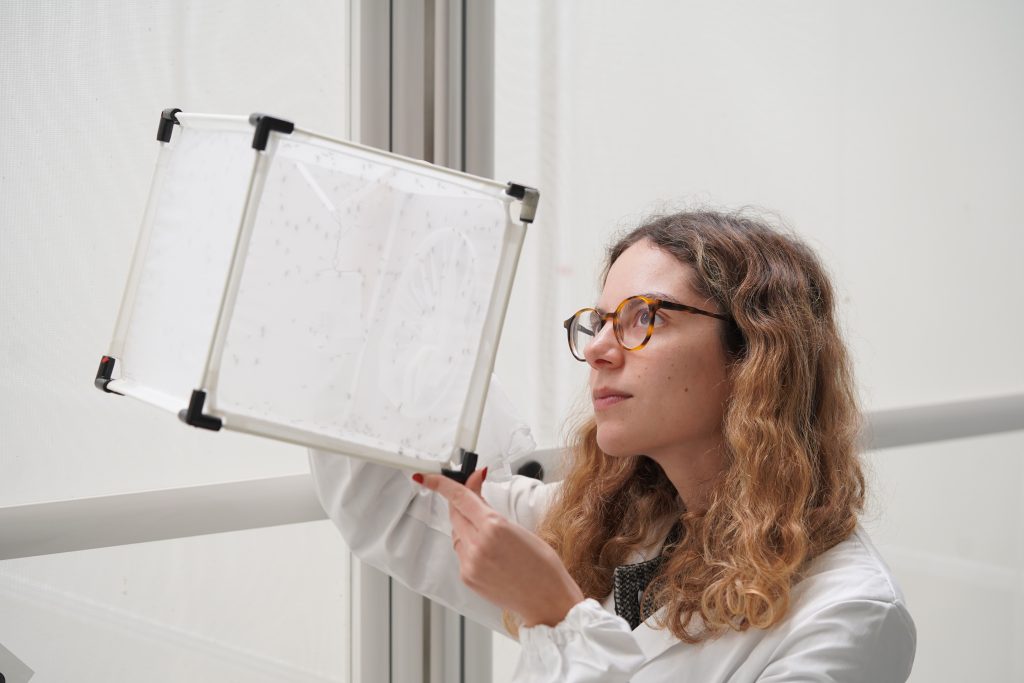
Malaria is one of the oldest diseases in the history of humankind, with references to it tracing back to 2000 BC. It is caused by Plasmodium parasites that are transmitted to humans by Anopheles mosquitoes. Though malaria used to be widespread around the globe, continuous use of insecticides against its mosquito vector reduced its prevalence.
Still, it remains endemic to sub-Saharan Africa, where it claimed over 600,000 lives in 2022, mostly children under the age of five. This is a stark 30 per cent increase in malaria deaths compared to pre-pandemic years! Current insecticide-based interventions have long been deemed insufficient to achieve malaria eradication alone.
My PhD at Imperial College London in the Department of Life Sciences focused on engineering novel and species-specific genetic control technologies called gene drives to reduce the population of the major malaria vector Anopheles gambiae.
What is a gene drive and how can we use it to fight malaria?
Gene drives are engineered selfish genetic elements, inspired from naturally occurring ‘selfish’ genes. We call them selfish because they promote their own inheritance to rapidly increase their frequency within a given population, often at the detriment of the population itself.
By adapting precise gene editing tech (CRISPR/Cas9) to work as a gene drive, we can spread desirable traits in a population of mosquitoes, such as parasite-killing genes that limit their ability to transmit malaria.
However, this is tricky to implement because the malaria parasites are very likely to evolve resistance against any parasite-killing gene. Instead, researchers at Crisanti lab are taking the alternative approach of spreading genes associated with reduced female fertility to suppress the population size of the malaria mosquito.
Like insecticides, this approach is aiming to reduce malaria transmission by tackling the insect responsible for spreading it. However, it is species-specific, as it targets unique DNA sequences and relies on mating to spread.
For example, there are over 3,500 species of mosquitoes, but only three species transmit most of human malaria. Using gene drives we can target the small handful of species that cause harm, without affecting other ecologically important species.
Moreover, gene drives are self-sustaining: the idea is that a small number of gene drive mosquitoes released in a natural population would be enough to spread the gene drive within that population, eventually causing its reproductive decline.
In 2018, a gene drive developed in the Crisanti Lab was demonstrated to spread in lab-contained populations of malaria mosquitoes, ultimately causing them to collapse. The gene disrupted the sex determination gene doublesex, which caused female mosquitoes to be sterile. Despite this success, as with any suppressive technology (e.g. insecticides, antibiotics, etc.), gene drives are prone to resistance.
Pre-empting this, my PhD focused on assessing the types of resistance expected to arise from gene drive implementation in the natural environment, and consequently on engineering more robust gene drives that mitigate said resistance.
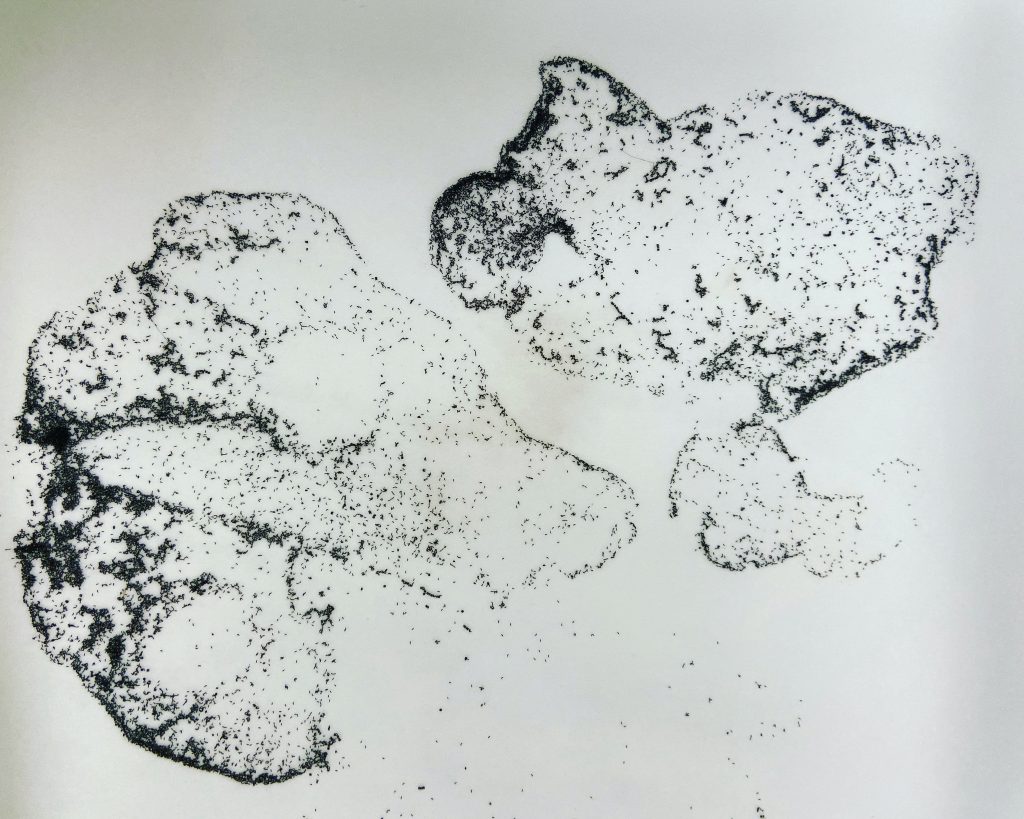
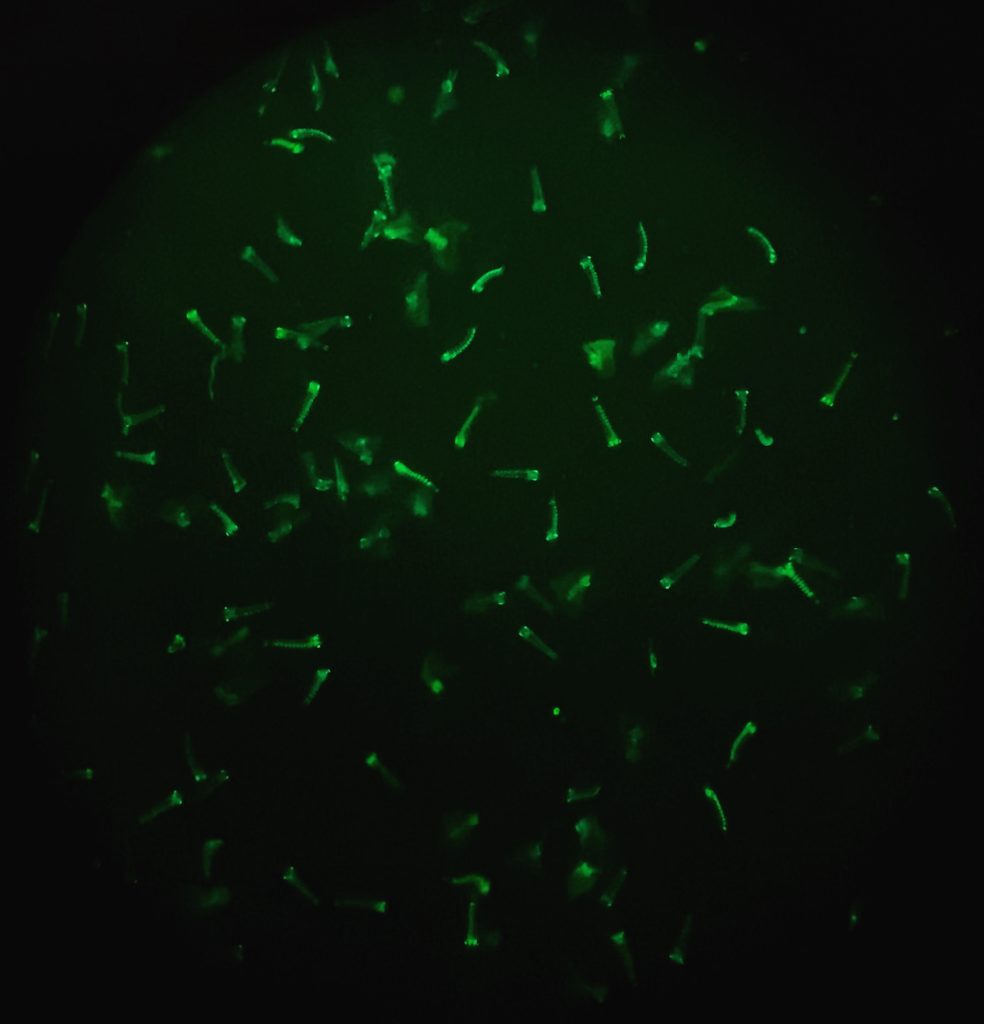
My personal journey
I first encountered gene drive research during my second year of undergraduate studies at Imperial, back in 2015. I was working on an extensive literature review of the field, when the first proof-of-principle that gene drives can suppress lab-contained populations of the malaria mosquito was published.
I found the different ways in which gene drives can be designed to cheat evolution and spread traits with an evolutionary disadvantage ingenious. That summer, in 2016 I joined Crisanti lab as a UROP student and was very lucky to be supervised by the people who developed the first functional gene drive. The following year, I secured funding to cover four and a half years of postgraduate studies, and after completing my MRes in Molecular and Cellular Biosciences, I began working on my PhD project at Crisanti Lab in 2018.
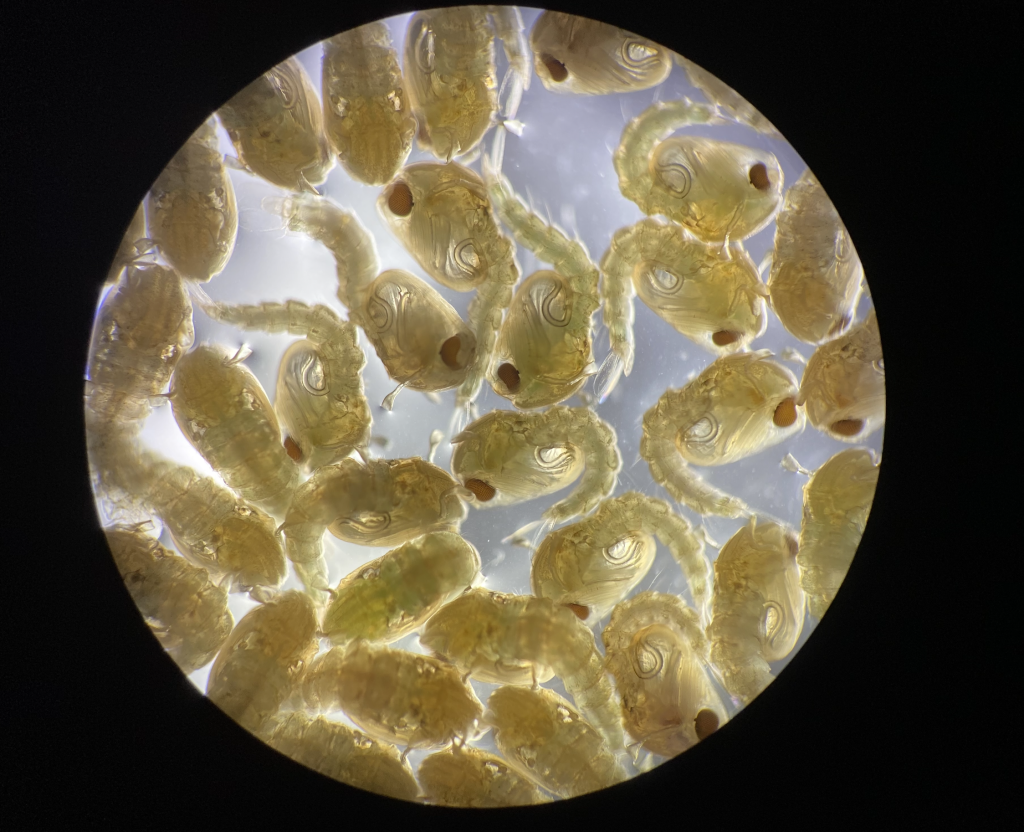
As with many of my peers, the biggest challenge I faced during my doctoral studies was the research interruption imposed by COVID-19 restrictions in 2020, which halted the most crucial part of my data collection.
Pre-pandemic, we were maintaining approximately 140 distinct transgenic mosquito stains that we use for experiments in our lab. We could only keep half of them after restrictions were in place, and all ongoing experiments, that might have taken several months to set-up, had to be killed. For about six months I did not make any progress. I was disheartened at the time, but looking back, I think it allowed me to better prioritise experiments and set clear goals towards completing my PhD after restrictions were lifted.
Eventually, I completed my research around May 2022 and embarked to present it in conferences, in California and Crete – the perks of being an academic – before settling into my new role in July 2022 as a research and development (R&D) team leader at Biocentis.
Biocentis is an Imperial College spin-out that seeks to develop self-limited genetic control technologies against non-malaria disease vectors and agricultural pests, that is co-founded by pioneers in gene drive research. Inspired by gene drive and leveraging what we learned from our work in the malaria mosquito, we aim to develop technologies that utilise similar molecular components to gene drive but are instead limited in their ability to spread in space and time, for local implementations.
In particular, I am leading a small team working on the spotted-wing fruitfly, Drosophila suzukii, which is an agricultural pest of soft-skinned fruit like cherries, raspberries and blueberries. This fruitfly is particularly damaging as it lays its eggs inside ripening fruit, in contrast to other species that only infest already rotten fruit.
The transition from academia to industry
Initially my transition was challenging, as I was juggling moving to a new country, being in a completely new role, and writing-up my PhD thesis! First, it was hard to let go of my previous research, as I am sure is the case for many PhD students, after having devoted three to four years of continuous hard work towards a single goal. However, starting work from scratch in a new model organism was very exciting.
I also had to develop new skills that extended beyond my R&D roles, such as being responsible for conducting interviews and recruiting personnel, networking and forming international collaborations, and being involved in writing grant proposals and patent applications, amongst others.
I had to learn how to work in a more streamlined fashion, follow detailed timelines and report updates on a much more regular basis, whilst actively learning how to be a good manager (which can be very different compared to supervising students).
The most positive surprise in my transition was the collaborative sentiment that I encountered within the company. We always support each other as needed, being connected by our common goal of developing products that will have a positive impact to society. At the same time, we are maintaining our links to academia, as plenty of fruitful collaborations can result from this type of partnership.
My advice to any recent graduate thinking about joining a company, whether interested in R&D or business development, is to try it out. The similarities and differences between industry and academia might surprise you, and only experiencing it yourself will help you decide what suits you best.