Postdoctoral researcher in the Space and Atmospheric Physics group, Adrian LaMoury, delves deep into the science of auroras and the future of forecasting space weather.
“Any chance of northern lights in Cambridge tonight? Saw a dubious tweet”
This was the message I received from a friend on the evening of 10 May 2024. I replied that it was their best chance in years.
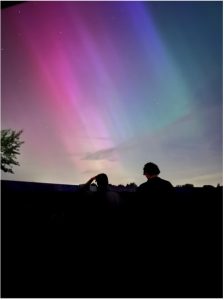
My inbox was blowing up with similar messages that night – emails from colleagues and notifications from aurora forecasting services. Something big was happening. Sure enough, stunning aurora photos began to emerge from Scotland and the North, then the Midlands, and eventually my friend in my Cambridge.
My partner and I dashed outside to see if anything was visible in the sky but we were disappointed (but not surprised, given we were on England’s south coast surrounded by light pollution, not exactly Svalbard). Instead, we went to a nearby bar where I lectured my friends on the physics of the aurora.
How come there were auroras in Cambridge?
We can only see the aurora in the darkness of night, but its origin is the Sun. Our solar system is full of plasma (a superhot ionised gas) that streams out of the Sun at hundreds of kilometres a second. We call this slew of plasma the ‘solar wind’, and its gusts can be felt all the way past Neptune and Pluto.
Like Earth, the Sun has a magnetic field, and it is far stronger than ours. The solar wind carries the Sun’s magnetic field and when it reaches Earth, the two fields cannot mix, repelling each other like two bar magnets.
Earth’s field therefore acts as a shield from the solar wind, carving out a protective bubble around us in space that we call the ‘magnetosphere’. The magnetosphere isn’t a perfect shield, however. Occasionally, pockets of solar wind slip through, trapping energetic particles in the Earth system. After a circuitous journey, some of these particles find themselves raining down on the top of Earth’s atmosphere.
The beautiful lights you see in the aurora are a result of emission from collisions with atmospheric gases, like oxygen.
There are particularly intense and fast solar wind events, known as ‘coronal mass ejections’ (CMEs). If they hit Earth, they readily penetrate the Earth’s magnetic defences. Auroral displays are usually confined to the polar regions, but in larger storms they drift equatorward. This is what happened on 10 May.
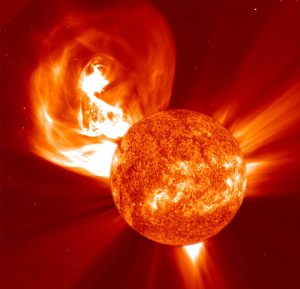
What’s the weather like up there?
Auroras are the most common and visible manifestation of what we call ‘space weather’. This is a wide-ranging set of phenomena we experience on Earth as a result of our magnetic connection with the Sun. Like terrestrial weather, it’s mostly nothing to worry about. Every now and then, however, space weather can have profound consequences for society and infrastructure.
All electrical systems and technology are sensitive to magnetic fields, as a varying magnetic field can induce a current. The geomagnetic disturbances produced by space weather events can therefore be hugely disruptive to power grids, in extreme cases even leading to transformer harm and widespread outages.
Satellites are particularly vulnerable because energetic particles can damage circuitry, cause irregularities in transmissions, and even force re-entry for those in low Earth orbit. We saw this in February 2022 when 38 Starlink satellites came crashing back to Earth after launching during the impact of a CME.
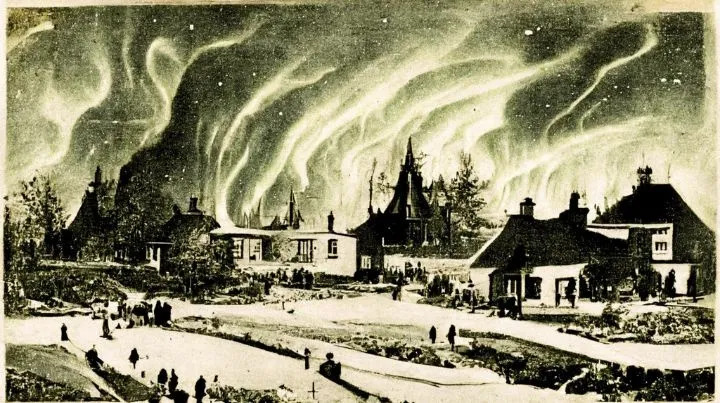
The most famous, and most powerful, space weather event in recorded history occurred in 1859. A very large and fast CME struck the Earth, producing auroral displays the world over – reportedly as far south as Mexico and Cuba. Along the electrical telegraph network, there were reports of operators receiving shocks and fires breaking out at stations due to the huge currents induced in the cables and the ground. Now known as the ‘Carrington Event’ after British astronomer Richard Carrington who documented the onset, the storm of 1859 is often used as the benchmark for a worst-case scenario.
A Carrington-scale CME impact today would likely be catastrophic, with worldwide blackouts and almost complete loss of digital communications lasting anywhere from hours to weeks. Estimates of the global cost of such an event are in the trillions of dollars.
Mercifully, events of this magnitude are extremely rare, but we can start taking steps to make our infrastructure more resilient to space weather, as well as developing forecasting capabilities to give us advance warning of significant impacts.
Over the last decade, expertise from academia and industry has been compiled into the UK Severe Space Weather Preparedness Strategy. One of the outcomes of the strategy was the Space Weather Instrumentation, Measurement, Modelling, and Risk (SWIMMR) programme, a £20 million funding scheme that ran from 2019 until earlier this year, with the goal of improving the UK’s space weather monitoring and prediction capabilities.
Preparing for bad weather
Here at Imperial, we are part of the SWIMMR Activities in Ground Effects (SAGE) consortium. Alongside partners at the British Geological Survey (BGS), the British Antarctic Survey, and UCL, we have been developing forecasting products relevant to the UK power grid, rail network, and high-pressure gas pipelines.
Imperial’s contribution to SAGE was a simulation code, called GorgonOps, which models Earth’s magnetosphere and how it responds to changes in the solar wind.
GorgonOps is the result of over a decade’s development by many scientists across Imperial’s Department of Physics. Its original purpose was to model laboratory plasmas, the kind used in nuclear fusion experiments. It was dubbed ‘Gorgon’ as the writhing tendrils of plasma in the simulation bore resemblance to the serpentine hair of Medusa.
Gorgon was later adapted to model space plasmas and has been used very successfully to probe the magnetospheres of Earth and Neptune.
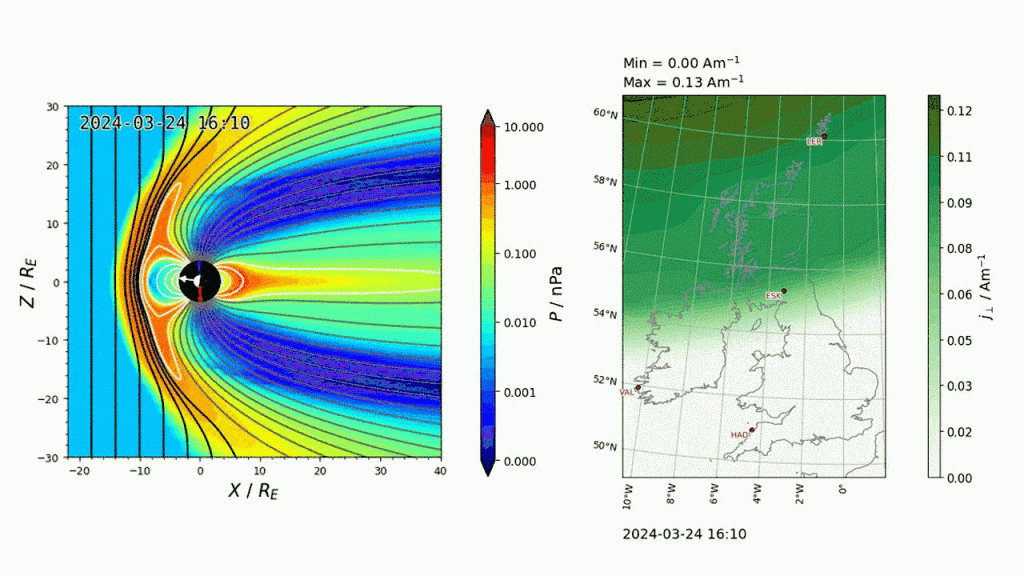
At every point in space in the simulation, Gorgon solves the magnetohydrodynamic (MHD) equations to understand how the plasma flows and magnetic fields evolve. Doing this takes an enormous amount of computational power, often requiring several days of supercomputer time to run.
This, of course, is not practical for space weather forecasting, where we need to make predictions as quickly as possible. Much of the development work of the last few years has therefore gone into slimming everything down in an effort to reduce runtime.
While you can get some of the way by simply optimising your code, it’s just not possible to capture all the physics in its fullest glory in the timescales we need with currently available technology. Some concessions had to be made. This included dropping the simulation resolution in some areas while retaining it in those most important to the forecasting.
At every step, the outputs were rigorously tested to ensure that they were still valid while making everything as lightweight as we could get away with.
All of this resulted in a code that runs in faster than real time, that is, the simulation moves quicker than the magnetosphere itself. Satellites sitting between Earth and the Sun give us near-constant measurements of the solar wind, which we use as simulation inputs.
We can do this continuously, making Gorgon fully operational for forecasting. We therefore call this version GorgonOps, and this is what sets it apart from other MHD magnetosphere models.
In the context of SAGE, we predict the changes to the magnetic field on the ground. These data are then fed into other models run by BGS, which map the ground conductivity across the UK, and give us an indication of the stress being placed on crucial infrastructure by space weather. This forecasting service is currently being integrated with the Met Office.
We can predict the possible effects up to an hour before they actually happen. This buffer means that, in the event of something serious coming, there is some time to issue warnings to industry and government so that they can take appropriate action. This could include disconnecting transformers from power grids so that they are not damaged by a surge and placing satellites into a safe mode to prevent irregularities.
My role, having joined the team as a postdoc last year, involves the general upkeep, testing, and development of GorgonOps. I’m responsible for benchmarking the outputs to ensure everything behaves as expected according to theory and measurements.
I’m also working on a project, in collaboration with the University of Bergen, in which we are developing a new space weather forecasting service for the European Space Agency (ESA).
Long range forecast
While enormous progress has been made over the last decade, we still have a long way to go until we are fully prepared for extreme space weather. As far as forecasting is concerned, the more advance warning we can provide, the better our chances of safeguarding our infrastructure.
This may come with new spacecraft such as Vigil, an ESA mission scheduled to launch in 2031, whose purpose is to monitor the Sun and solar wind from side-on, giving us a view of CMEs and the like before they are pointed at Earth.
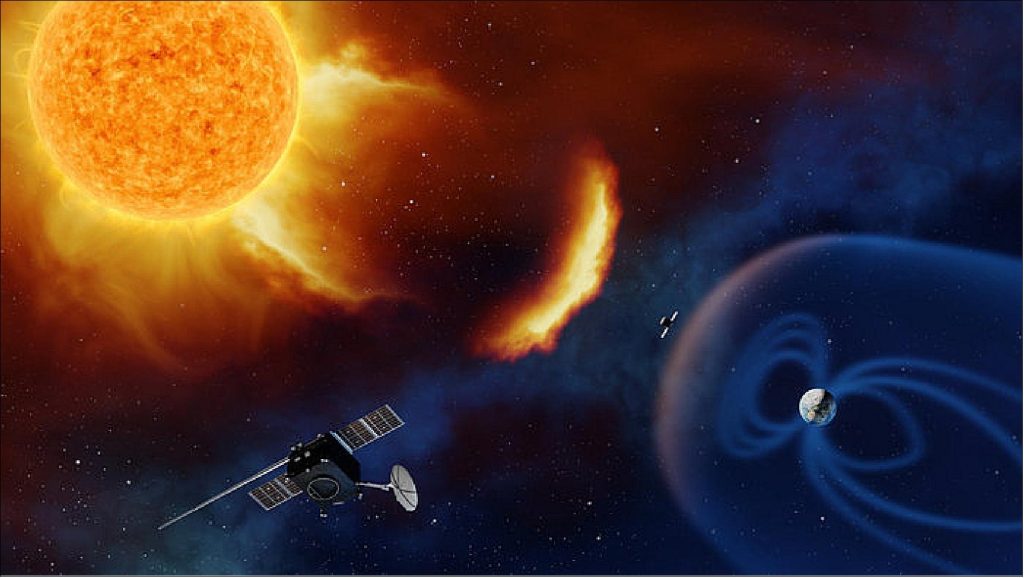
Imperial is heavily involved in building one of Vigil’s instruments, a magnetometer. As well as ensuring that the instrumentation is robust and reliable enough to provide a 24/7 data stream back to Earth, much of the work over the coming years will be in preparing our forecasting regimes to handle the new data.
As the world becomes increasingly reliant on technology, we also become increasingly vulnerable to space weather. Part of the preparation process is also raising awareness amongst the public, government, and relevant industries so that we can, as a community, make sure that we’re ready.