PhD student Harsh Rathee explores an experiment from Imperial’s Quantum Measurement Lab, which captures the peculiar nature of the quantum world.
Quantum physics is captivating yet perplexing, contrasting sharply with our everyday experiences. The allure and mystery surrounding the term “quantum” has unfortunately led to misconceptions in the general public. Hollywood has capitalised on this mystery, portraying quantum physics as the basis for instantaneous teleportation, time travel, and multiple dimensions.
While some of these explanations contain a kernel of truth, they often rely on a leap of faith to explain groundbreaking scientific advancements. However, it is important to note that the real-world progress in quantum technology is far from dull.
In recent years, there has been a surge in interest and development of quantum technologies. For instance, while teleportation of large objects is not practically possible, the application of quantum teleportation has transformed the field of quantum-information processing in a practical way, and scientists have already demonstrated the teleportation of quantum particles over extended distances.
Another technology of immense interest is the development of quantum computers. These computers possess capabilities far beyond those of current classical computers. They could potentially break common cryptographic protocols, solve complex mathematical problems, and efficiently simulate complex multi-particle quantum dynamics. These capabilities, in theory, could revolutionise fields such as drug discovery and protein structure analysis.
Quantum measurement at Imperial
Amidst this exciting landscape, I pursue research in Imperial’s Quantum Measurement Lab, led by Michael R. Vanner. The lab focuses on harnessing quantum measurement techniques to enable exciting applications, including engineering specific quantum states of matter, the construction of a quantum memory, and the exploration of the very fundamental physics such as exploring whether there are limits to existing quantum mechanics.
One recent study conducted by the team highlights the counterintuitive nature of quantum-scale measurements and their impact on the underlying systems. The work focuses on cooling a system by shining laser light on it. Cooling a sample using light usually works by sending a stream of light particles (photons) at the sample. The photons interact with the material and absorb energy in the form of vibrations, reducing the temperature of the sample in the process.
In this study however, light was directed into something called a microsphere – imagine a tiny, spherical cavity. We use it to trap light by letting it bounce inside a circular path, allowing us to confine light with very low loss.
We observed that measuring the absence of light emerging from the microsphere resulted in a drop in the sphere’s temperature greater than the conventional laser cooling techniques. In other words, we were able to cool a system with nothing more than the act of measuring it.
So how does this work?
In usual laser cooling, the photons enter the sphere and interact with the vibrations in it. Photos gain energy from the vibration, taking it away and cooling the system.
But in this case, we weren’t just observing the light that did come out — we were especially interested in the observations when no light was emitted at all.
In the quantum world, detecting a photon (a particle of light) confirms the system had enough energy to emit it. But if no photon is detected, it suggests the system was in a lower-energy, cooler state.
By carefully selecting and focusing on just these “no-photon” moments — a technique called post-selection — we find the system in cooler and cooler states. The result was a drop in temperature that went beyond what normal laser cooling could achieve.
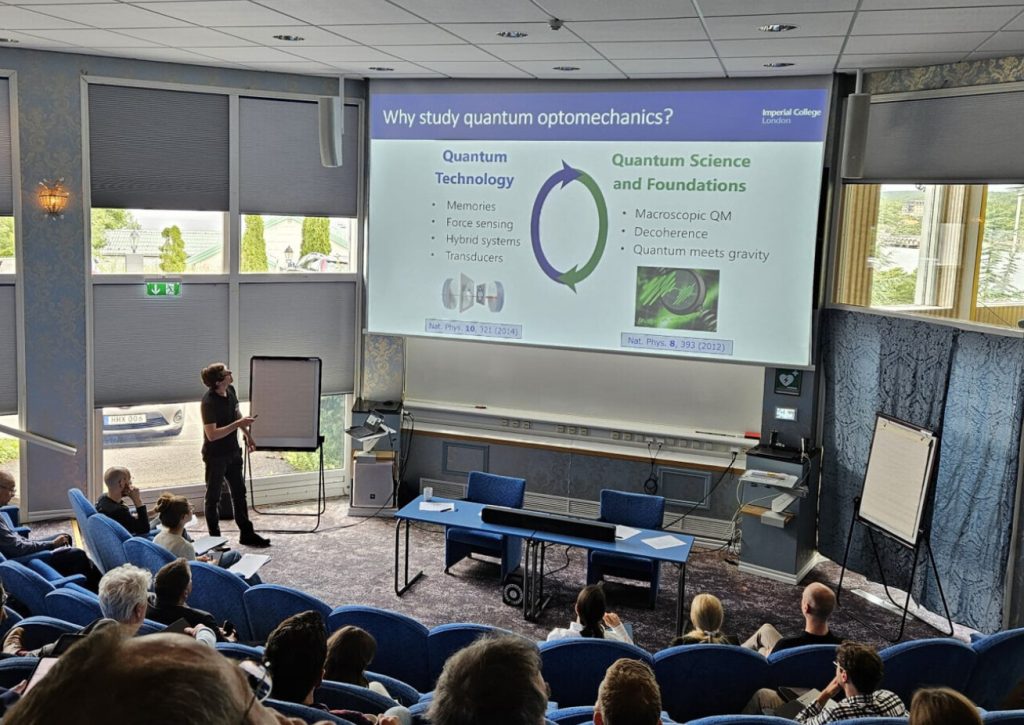
This shows the fascinating properties of quantum scale measurements on non-deterministic systems! Here, we’re not adding or removing energy directly, but we gain control over the temperature of the system by narrowing possible outcomes.
While cooling using lasers is a well-established technique in optical and atomic physics, conditioning the cooling process on the absence of light enables greater control over creating devices capable of operating in the quantum regime.
Excitingly, this work was featured by New Scientist and made it as a sub-title on the cover of the print magazine.
Quantum futures
This study builds on previous works by the team that demonstrated that measuring a single photon caused the temperature of the object to rise. This seems counterintuitive because when measuring a scattered photon, one expects the photon to have interacted with the material, extracting energy from the system, resulting in a colder sample.
Similarly, it is surprising that measuring the absence of something, in this case, light, has a tangible effect on the underlying system, causing it to cool. This apparent mystery is solved when one understands that the amount of vibrational energy in the sample is not deterministic.
It has, in fact, an underlying probability distribution and it is our lack of knowledge of the actual state of the system that gives it an average temperature. When a measurement is made (either of a single photon or no photon at all!), we update our information about the distribution of energy in the sample.
This technique of statistical inference is known as Bayesian inference. This peculiar phenomenon was covered in an Imperial news article, where we see that even experienced researchers are sometimes caught off guard by the strange occurrences in quantum experiments!
I joined Michael Vanner’s lab as a MSc student, captivated by the same peculiarities of quantum mechanics. My vision was to comprehend and harness this peculiarity to fuel the quantum revolution.
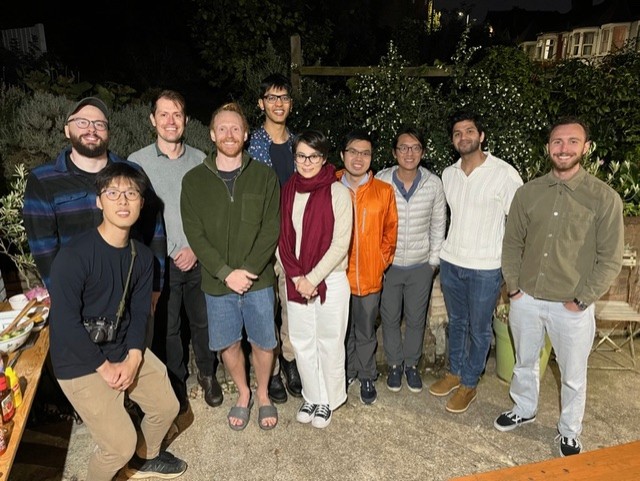
After nearly three years as a PhD student, I’ve gained valuable insights from my colleagues and explored the vast quantum landscape. While I’ve made significant progress, I recognise that there’s still much I don’t understand and numerous mysteries to unravel.
Despite these challenges, I remain optimistic about the future. The rapid advancements in the field over the past few years, coupled with the collective brilliance of researchers worldwide, instills in me a sense of hope.
I envision a future where we can harness the power of quantum technology to revolutionise our understanding of the world and propel our research and industries into the quantum era.